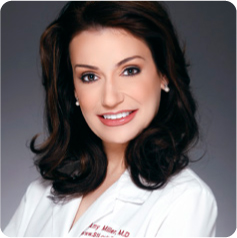
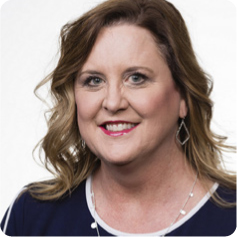
The use of ultrasound to image the skin and subcutaneous structures is a relatively new and increasingly prevalent tool that is available to the aesthetic practitioner. It has multiple applications within a cosmetic practice. Ultrasound can detect and measure signs of photoageing caused by prolonged exposure to the sun through the observation of the subepidermal low echogenic band (SLEB) (Alfagame et al, 2021). The SLEB is a hypoechogenic or dark band just below the epidermis, representing water and increased glycosaminoglycans in photoaged skin.
Prior to cosmetic injections of neurotoxin or dermal filler, ultrasound can be used to visualise the anatomy of a targeted area and screen for vessels and anatomic anomalies. Additionally, it can be used to characterise complications of filler injections, for example, granulomas, biofilms or delayed onset nodules. Ultrasound can be used to guide aesthetic injections or any therapeutic injections, such as hyaluronidase (Weiner, 2021), collagenase, steroids or deoxycholate. Additionally, it can be used to evaluate the placement of previous fillers, as well as threads (Lee et al, 2020). Ultrasound can be a useful tool to gauge the improvement of lipolytic treatments or body sculpting treatments by measuring subcutaneous adiposity and muscle thickness (Kilgariff, 2020).
Ultrasonography is especially useful in aesthetic injection emergencies. It can be used to evaluate blood flow and to look for filler-related vascular obstruction or occlusion. Once the obstruction is located, it can then be used as a guide for the injection of reversing agents, as well as visualisation of dissolution of the blockage and re-establishment of blood flow. This use alone is a compelling reason to overcome any barriers to ultrasound adoption in the aesthetics arena. Unfortunately, becoming proficient and competent with dermal and subdermal ultrasound can be a daunting and time-consuming task, especially for injectors who have not used sonography in their past training. The purpose of this paper is to provide basic information and tips for those who are beginning their journey with aesthetic ultrasound.
Ultrasound technology
The terms ‘ultrasound’ and ‘sonography’ tend to be used interchangeably. Ultrasound is a modality that uses acoustic energy or sound waves above the range of the human ear to create a two-dimensional image of varying shades of grey, black and white. The sound waves, originating from the probe of the ultrasound, penetrate the target human tissue and get progressively weaker as they are absorbed, scattered or reflected (Schelke et al, 2018). Reflected waves are picked up by the probe and displayed in a digital image, the degree of greyness in the image corresponding to the echogenicity of the tissue. For example, bone tissue, which has high echogenicity and reflects most of the sound waves back to the probe, will appear white in the digital image (minimal to no grey). However, water or hyaluronic acid absorbs the sound waves efficiently, leaving nothing to be reflected to the probe and appearing black. The varying shades of grey between these two extremes correspond to the echogenicity of the tissue and, by extension, the densities of the tissues. The darker the structure, the higher the water content, and the whiter the structure, the lower the water content.
Sound waves are measured in decibels (height of the wave that corresponds to the perceived loudness or intensity), as well as hertz (Hz), which is the number of oscillations of waves per unit of time. One hertz is equivalent to one oscillation per second, while one megahertz (MHz) is one million oscillations per second. In the case of ultrasound, the human ear cannot detect the high rate of oscillations of the sound waves, rendering decibel measurement moot. Ultrasonography in medicine generally uses anywhere from 1 MHz to more than 20 mHz probes. The higher the frequency (MHz), the better the picture resolution, but the poorer the penetration. Thus, for imaging skin and small, superficial structures, higher frequencies are better, in the 10–22mHz range. Deeper structures, such as organs and foetuses, are better imaged with lower MHz probes.
Colour and power doppler are modes that are used to assess the vascular anatomy and flow within vessels of the targeted tissue. Doppler technology is possible because of the principles of the Doppler effect. The Doppler effect describes the change in the pitch of a sound when the source of sound waves is moving relative to a stationary observer. The classic example is the change in the sound of an approaching and passing train whistle that is heard by a passenger waiting on the platform. In the case of blood flow, the probe's (the stationary passenger) sound waves are reflected to the probe by moving objects (blood cells) and the rate of perceived change is interpreted as the speed of the blood flow.
In colour doppler, red and blue colours are used to highlight the blood flow in one direction or the other with regards to the probe's position, with red usually meaning blood flow is towards the probe and blue is away from the probe (Odak and De Jesus, 2021). Remember: these colours do not represent the arterial or venous flow. Brighter colour generally means faster flow and darker colour represents slower flow. Turbulent flow is represented by the mixing of blue and red within a vessel and, sometimes, represents an area of vascular spasm as occurs during ineffective flow during vascular occlusion (Weiner, 2021).
Power doppler displays the strength of the doppler signal, rather than flow velocity and directional information (Martinoli et al, 1998). It is more sensitive than colour doppler in detecting blood flow. It is particularly useful for evaluating blood flow in slow flow vessels or smaller vessels that are not captured well by colour doppler alone (Turetschek et al, 1999).
Ultrasound terminology
Ultrasound terminology is detailed in Table 1.
Table 1. Ultrasound terminology
Ultrasound term | Definition |
---|---|
Ultrasound | Uses sound waves of very high frequency (2MHz or greater) that are above human hearing. Key: The higher the frequency, the less depth of penetration. The highest frequencies provide the best image resolution. The lower frequencies are needed for penetration but may not be as detailed. |
Transducer or probe | Converts electrical energy into ultrasound energy for imaging. Consists of one crystal element that sends and receives the ultrasound signal. Needed to create an ultrasound image. |
Gel (couplant) | Medium needed to use between the skin of the subject and the face of the probe to transmit sound for imagining (if there is an air gap, no image will be produced). |
Anechoic | The structure has no echoes within it. It will appear black because it produces no echoes. This is seen with fluid-filled structures and vessels. |
Hypoechoic | Used to describe an area that is darker compared to the adjacent areas. It will have a decreased brightness. |
Hyperechoic | Used to describe an area that is brighter than the adjacent areas. It will have an increased brightness. |
Homogenous | The structure has a uniform appearance and echo texture |
Heterogeneous | The structure has a mixed appearance of varying echo texture. Not uniform. |
Shadowing | A sound beam cannot pass through a structure because it is so dense that the sound beam reflects back and cannot penetrate. This happens with structures such as bone or calcifications or air. Therefore, shadowing appears behind it. |
Enhancement | The brighter area seen behind a structure. This happens with fluid-filled structures, as the sound accelerates through the fluid to the tissue behind it. This may help you distinguish between cystic versus solid structures. |
Gain | Used to increase or decrease the brightness of the received echoes displayed (some systems have an auto gain to assist in optimal imaging). |
Focal zone | Focuses the sound beam into a smaller beam at that area. This is where the best images will be obtained. You want to place this at the level of the anatomy you are imaging. Referred to as the ‘sweet spot’. You will find the focus on the monitor (arrow) on the side of the image (some systems have an autofocus that may assist you). |
Ultrasound anatomy
The ability to visualise the different layers and vascular structure of the human face in real time is one of the greatest advantages of working with facial ultrasound. When injecting in a high-risk area, such as the pyriform fossa, lips or glabellar region, the main arteries in these regions can be identified and their depth measured. After injection, if there is any question of vascular compromise (skin colour changes or increased pain reported by the patient), the vessels can, again, be visualised and the flow assessed.
When starting with facial ultrasound, the instructors at Cutaneous Facial Ultrasound recommend starting at the jawline, with the transducer close to the ear to visualize the parotid gland (Velthuis and Schelke, 2022). Sliding the transducer forwards toward the mouth, the masseter muscle can be identified in front of the parotid gland, as well as the superficial musculoaponeurotic system (SMAS) and facial artery (Figures 1 and 2).
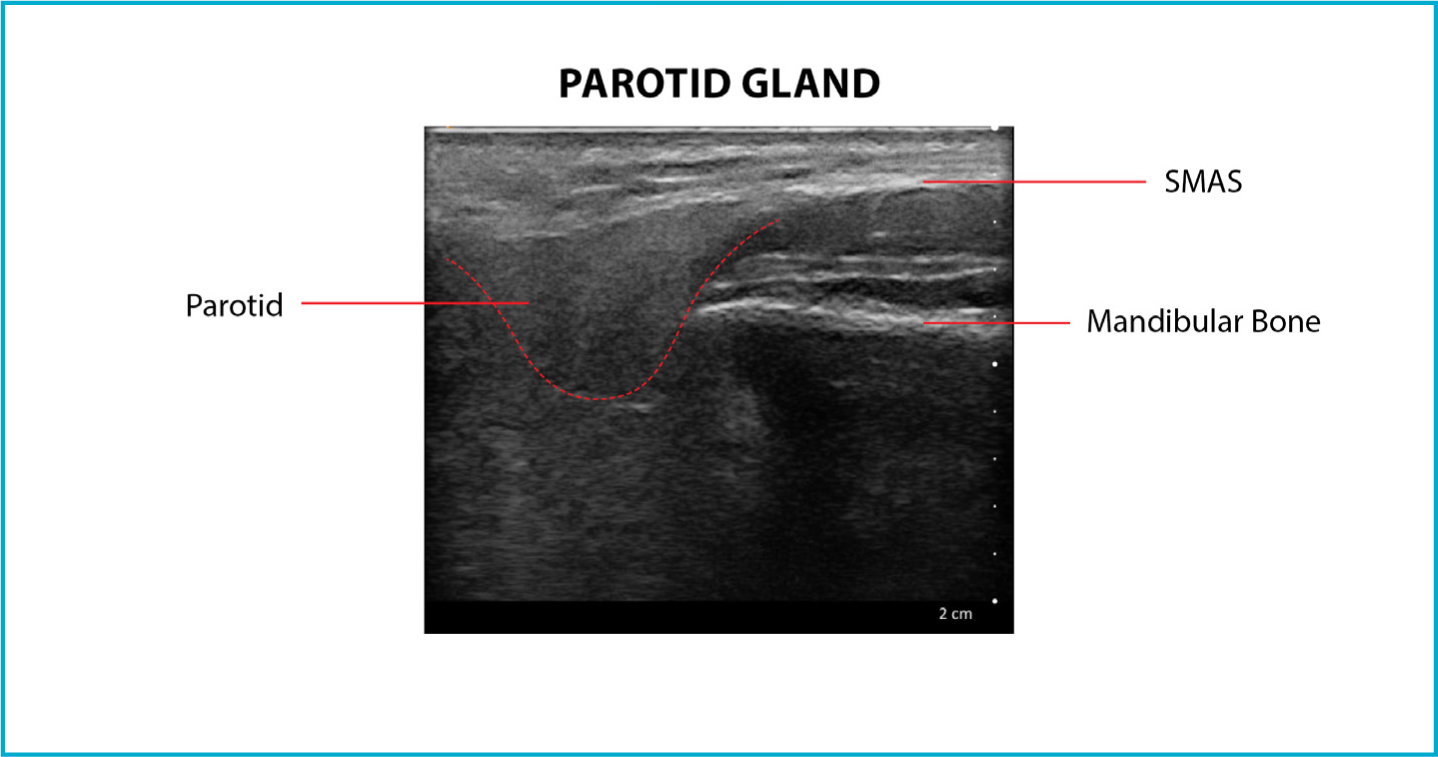
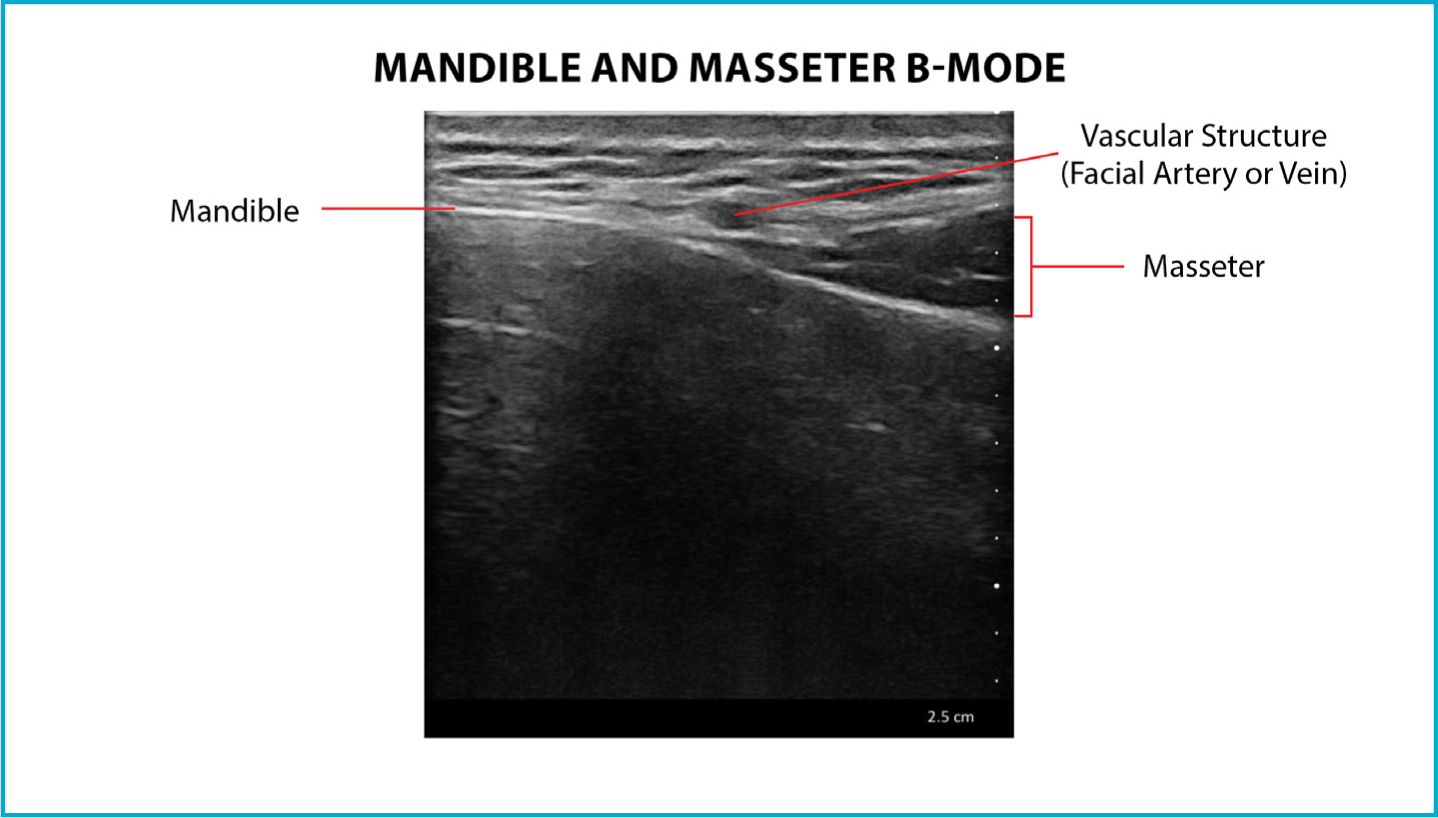
Switching from B mode to colour doppler, the facial artery can be readily discovered (Figure 3). Following the artery inferiorly, it can be seen crossing the mandibular border on its ascent from the external carotid artery (Figure 4). It is at this crossing point that the facial arterial pulse can often be palpated.
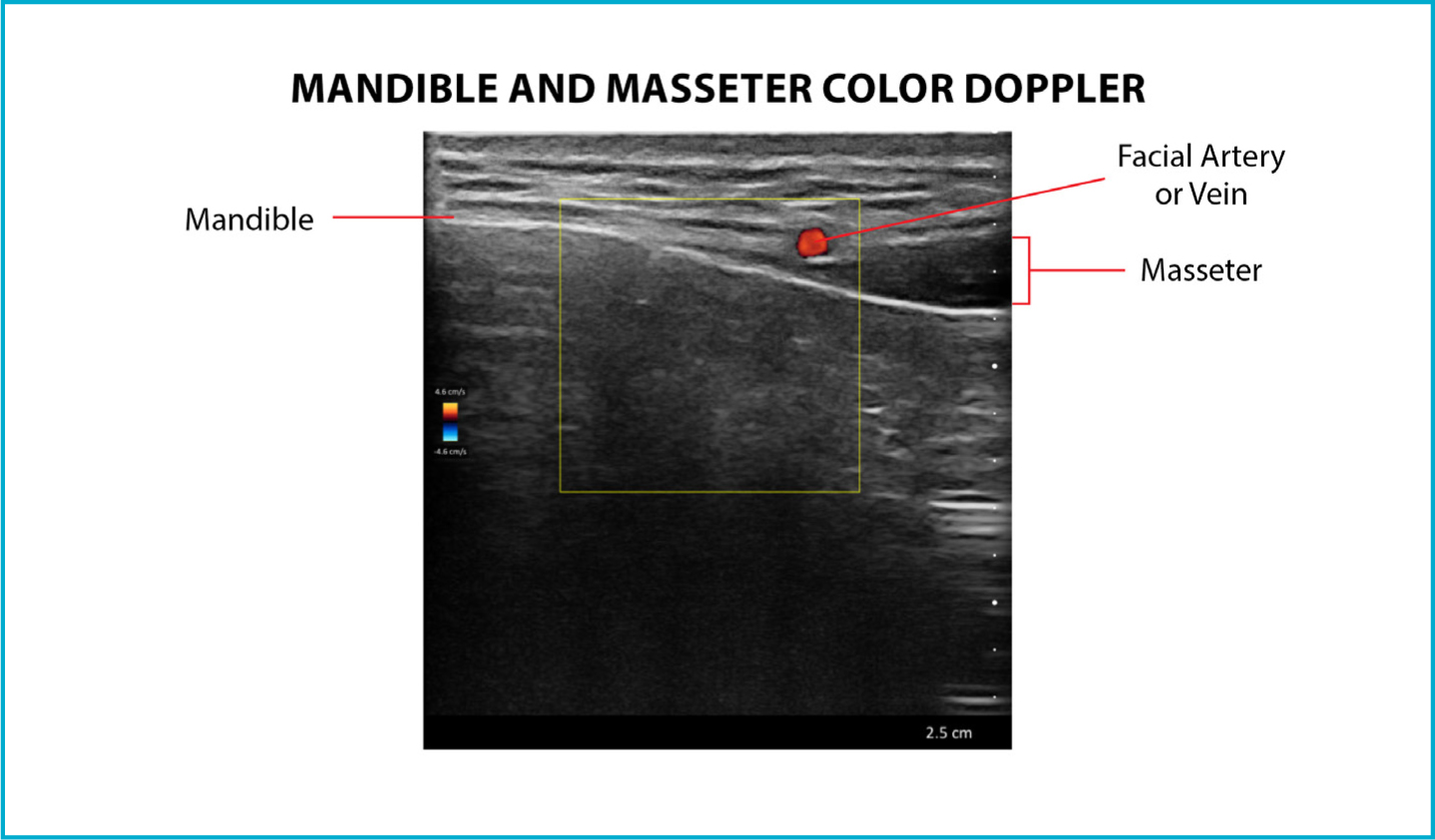
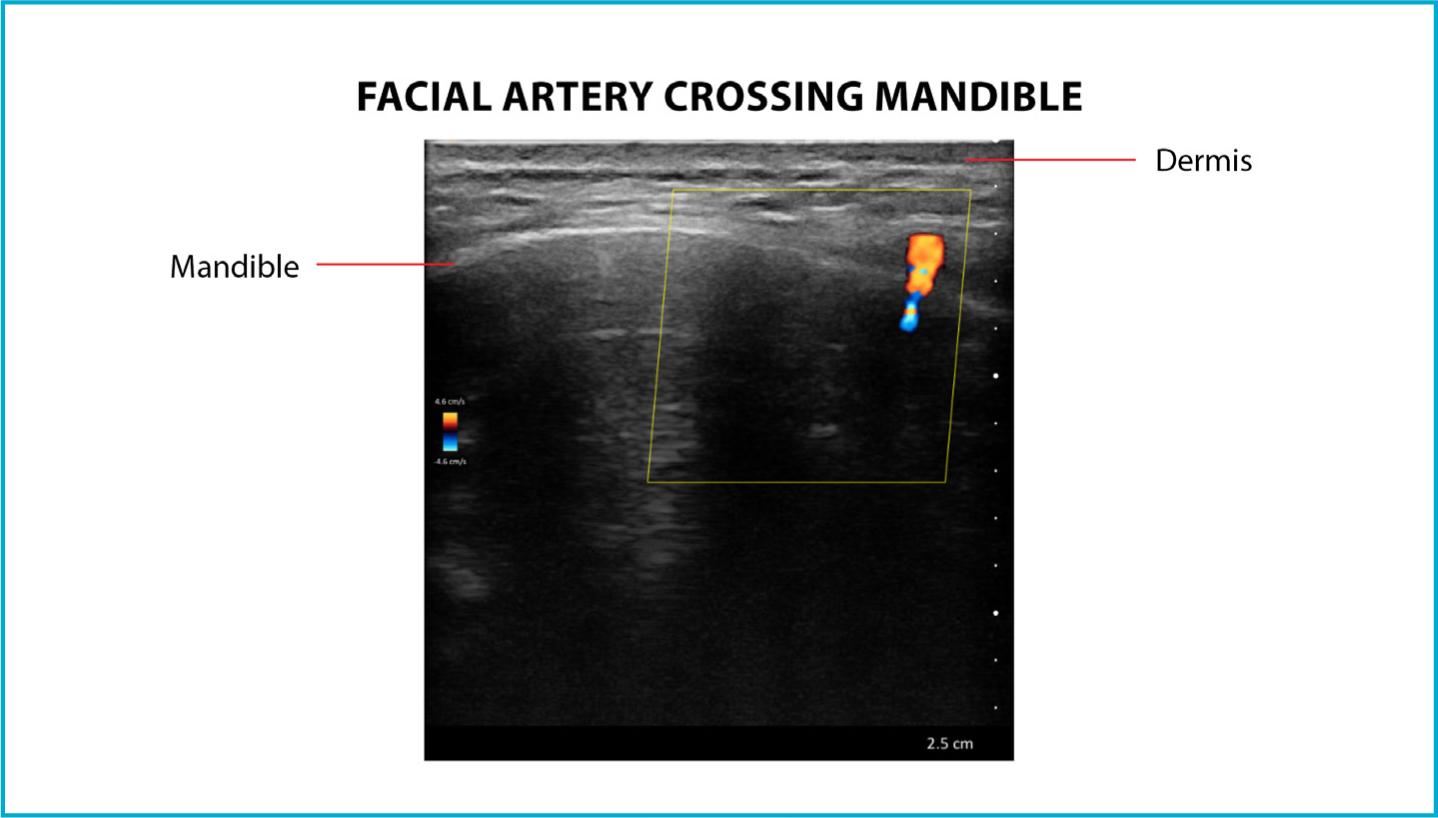
Moving medially to the chin, the depressor angularis oris (DAO) can be seen superficial to the depressor labii inferioris (DLI), with the mentum at the base (Figure 5). This image can be helpful when treating patients with neurotoxin in the lower face. Inhibiting the DAO can result in a subtle, pleasing lift of the oral commissures. However, inadvertent treatment of the DLI can result in a displeasing asymmetry of the smile. If treating the DOA at its midpoint, this image makes it obvious why it is important to stay superficial with the injection.
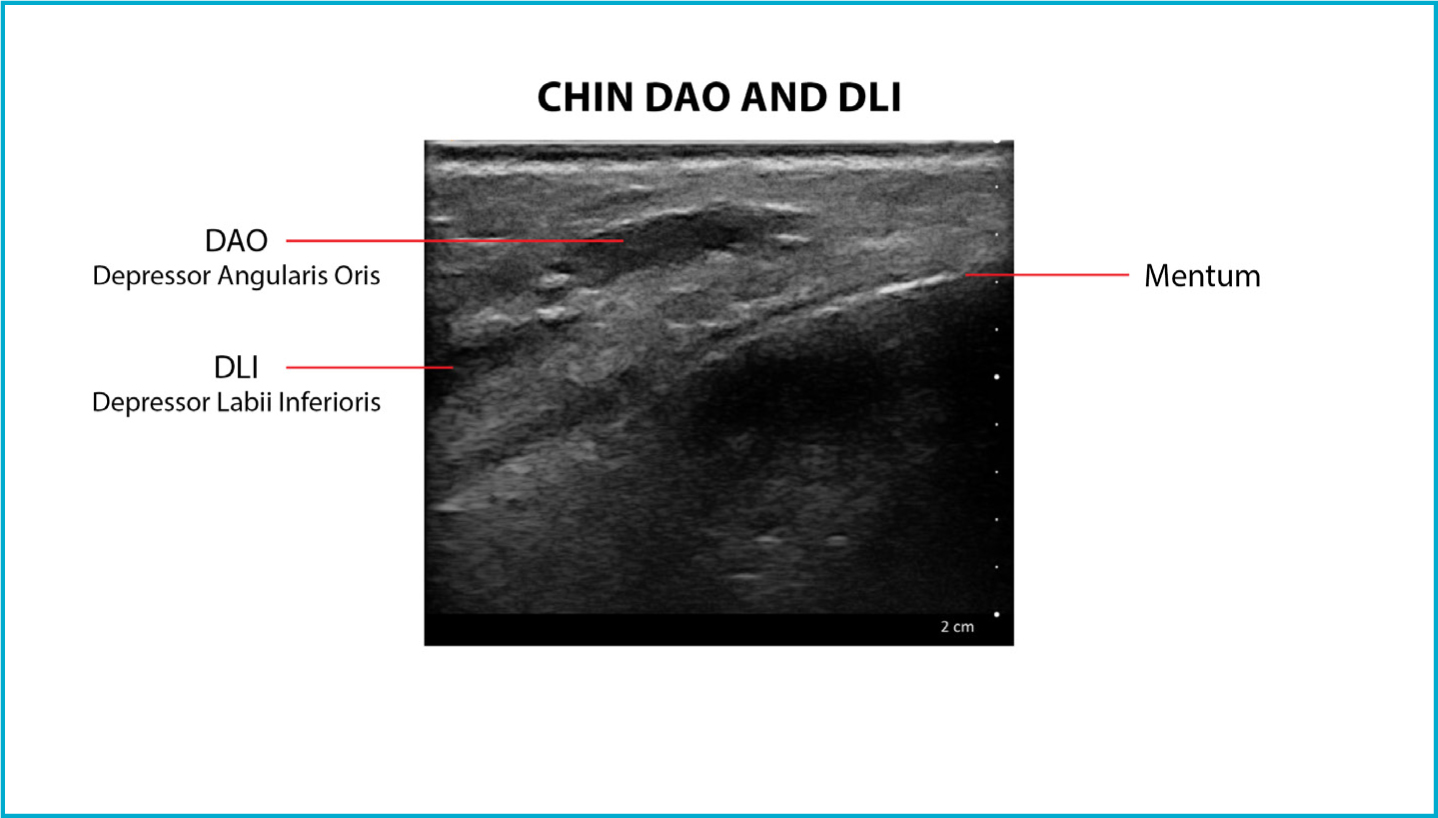
Moving to the upper lip, the orbicularis oris can be identified between the oral mucosa and the subcutaneous tissue and dermis of the cutaneous upper lip (Figure 6). The teeth can also be readily seen. The superior labial artery (SLA), inferior labial artery (ILA) and labiomental arteries branch from the facial artery and are the main arterial supply of the lips. There is substantial variation in the anatomical structure of this subunit, and even variation in the individual from one side of the face to the other. Anatomists have classified the most common variations into types I–VIII (Al-Hoqail and Meguid, 2008). Regarding the SLA alone, it has been found to run in the submucosal plane in 76% of cases, intramuscularly in 24% of cases and subcutaneous in less than 1% (Samizadeh et al, 2019), but it is known to change planes in 29% of cases (Cotofana et al, 2017). Essentially, the course of the SLA can be unpredictable and presents a problem if injecting filler into the lips. The safest course of action may be to stay subcutaneous and not attempt deeper injections unless one can monitor blood flow in real time with ultrasound and assure no flow disruption.
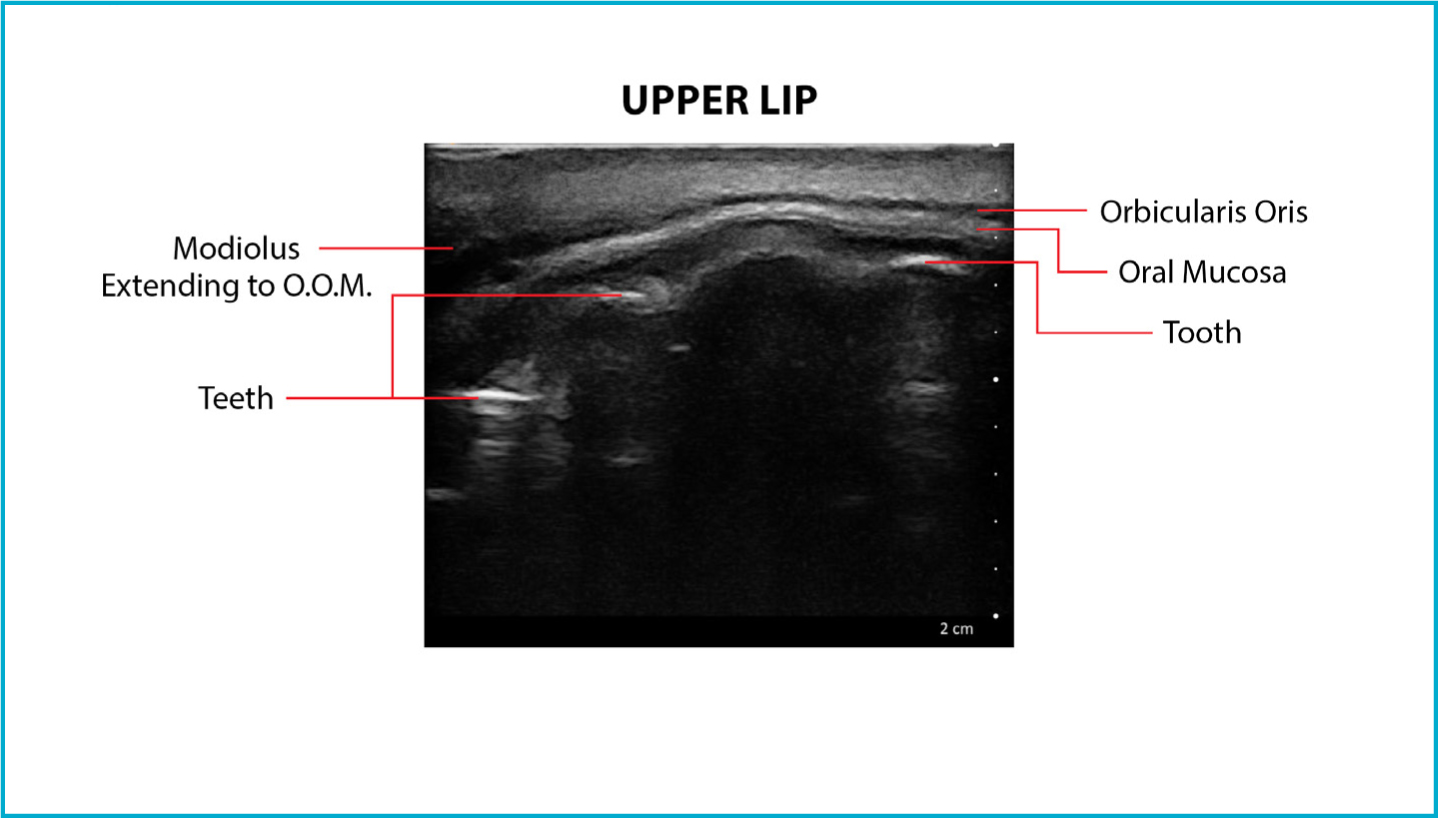
In addition to the lips, nose and glabella, a particularly dangerous area for injection of dermal fillers is the pyriform fossa and nasolabial fold (NLF) area. This is where the facial artery travels from the mandible toward the oral commissure and onto the nose. Close to the nare it changes course to travel north, along the base of the nose, as the angular artery (Figure 7). When the angular artery reaches the medial canthus, it can anastomose with the dorsal nasal artery and ophthalmic artery, as well as the supraorbital artery. Therefore, any filler injected inadvertently into the angular artery can reach the nose, eye and retina and forehead, potentially causing necrosis and blindness.
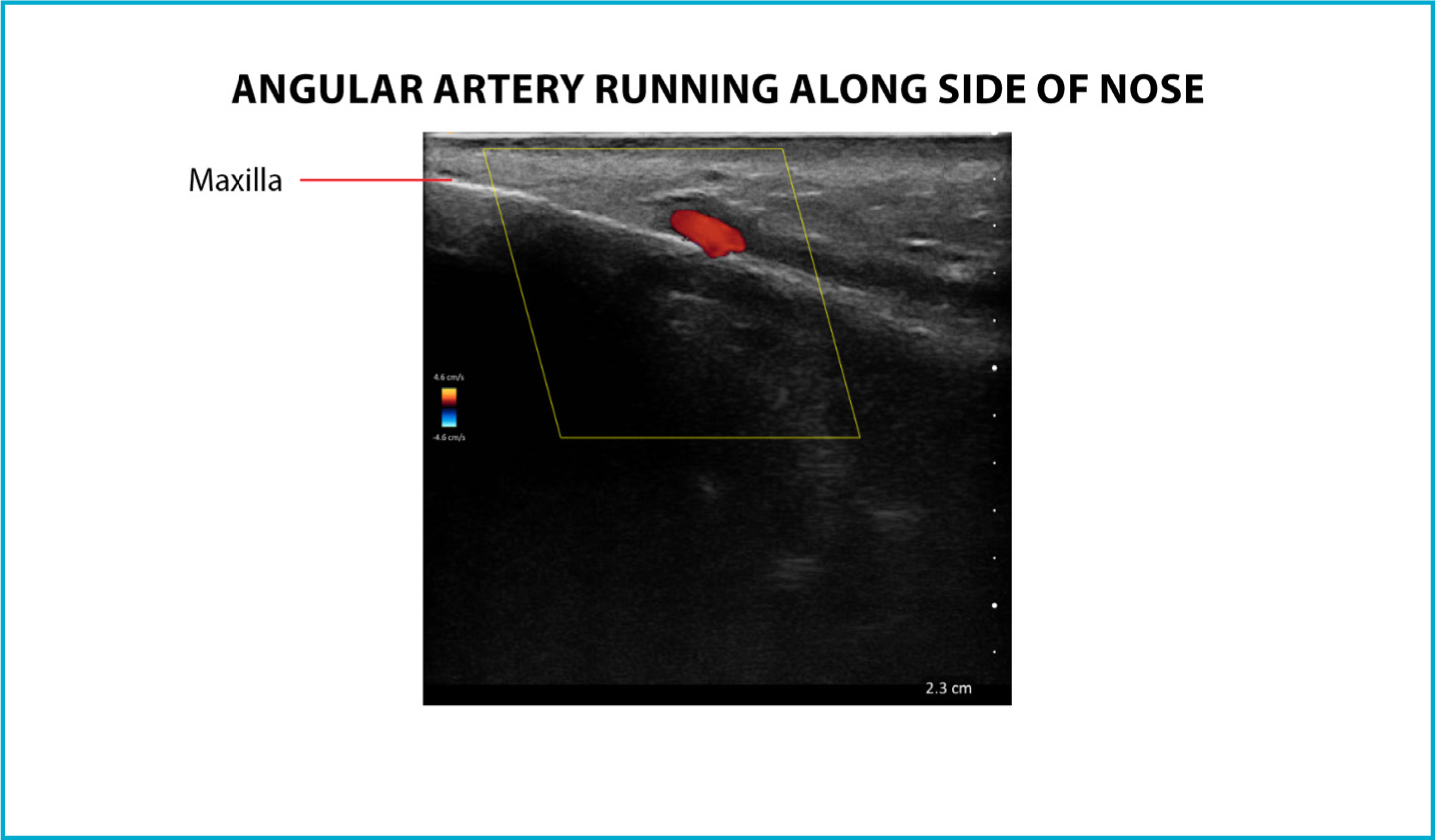
The facial/angular artery is especially vulnerable to penetration at the pyriform fossa. Filling this area can provide a pleasing anchor for filling the NLF and support the nose by stabilising ageing-induced widening of the nasal aperture. Visualising the angular artery in this location with U/S (Figure 8) can help the injector gauge the depth of the vessel pre-injection and reassure the injector after the injection.
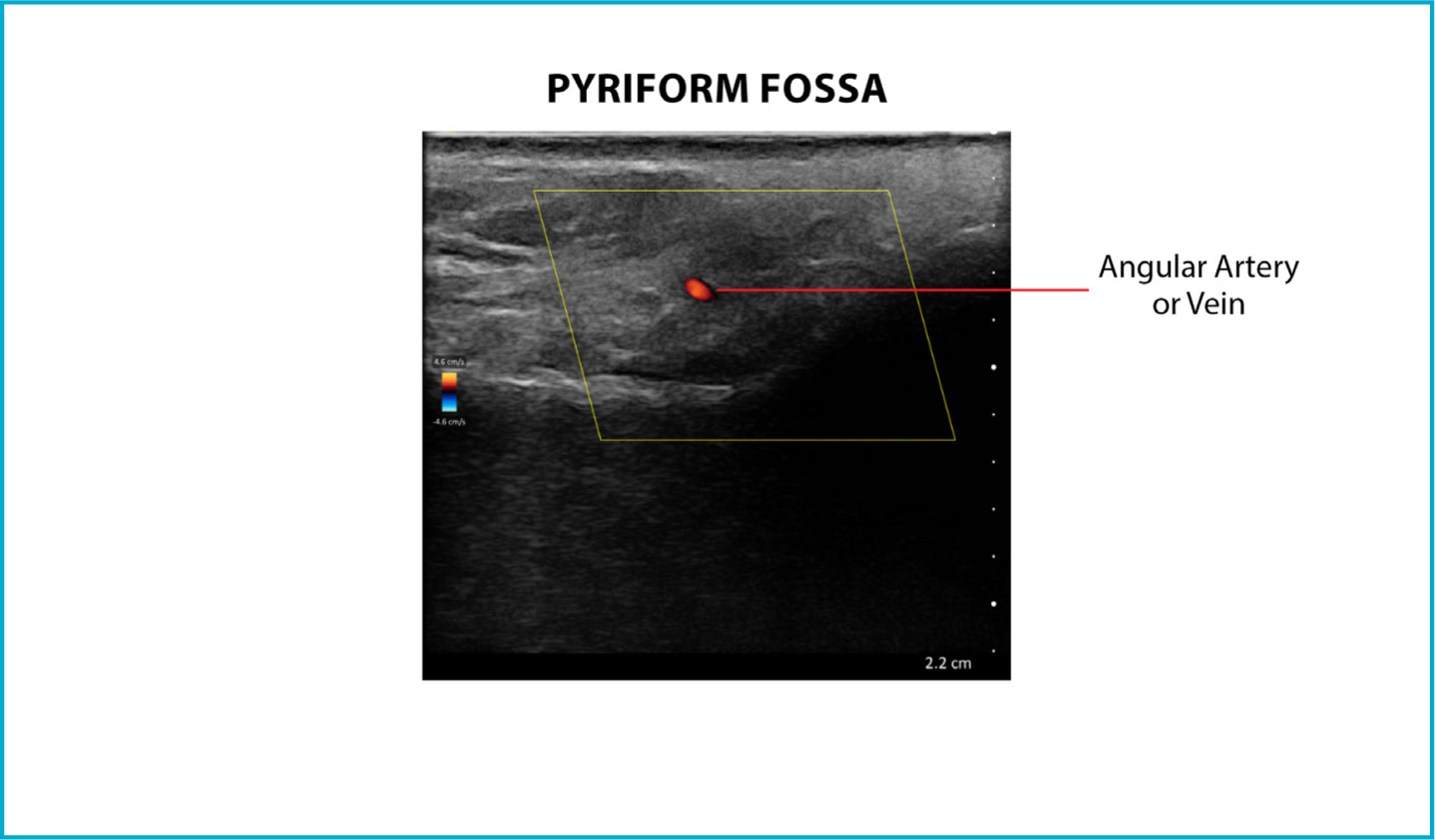
Sliding the transducer laterally from the nose to mid-cheek, the infraorbital artery can be seen emerging from the infraorbital foramen (IOF) (Figure 9). In 75% of cases, the IOF is located on the line that connects the lateral palpebral commissure to the ala of the nose (Ercikti et al, 2017). The infraorbital artery branches out and supplies the lower eyelid and lacrimal sac, the lateral nose and the mid-cheek and part of the upper lip. It anastomosis with the angular artery and dorsal nasal artery, as well as the ophthalmic artery, in most cases.
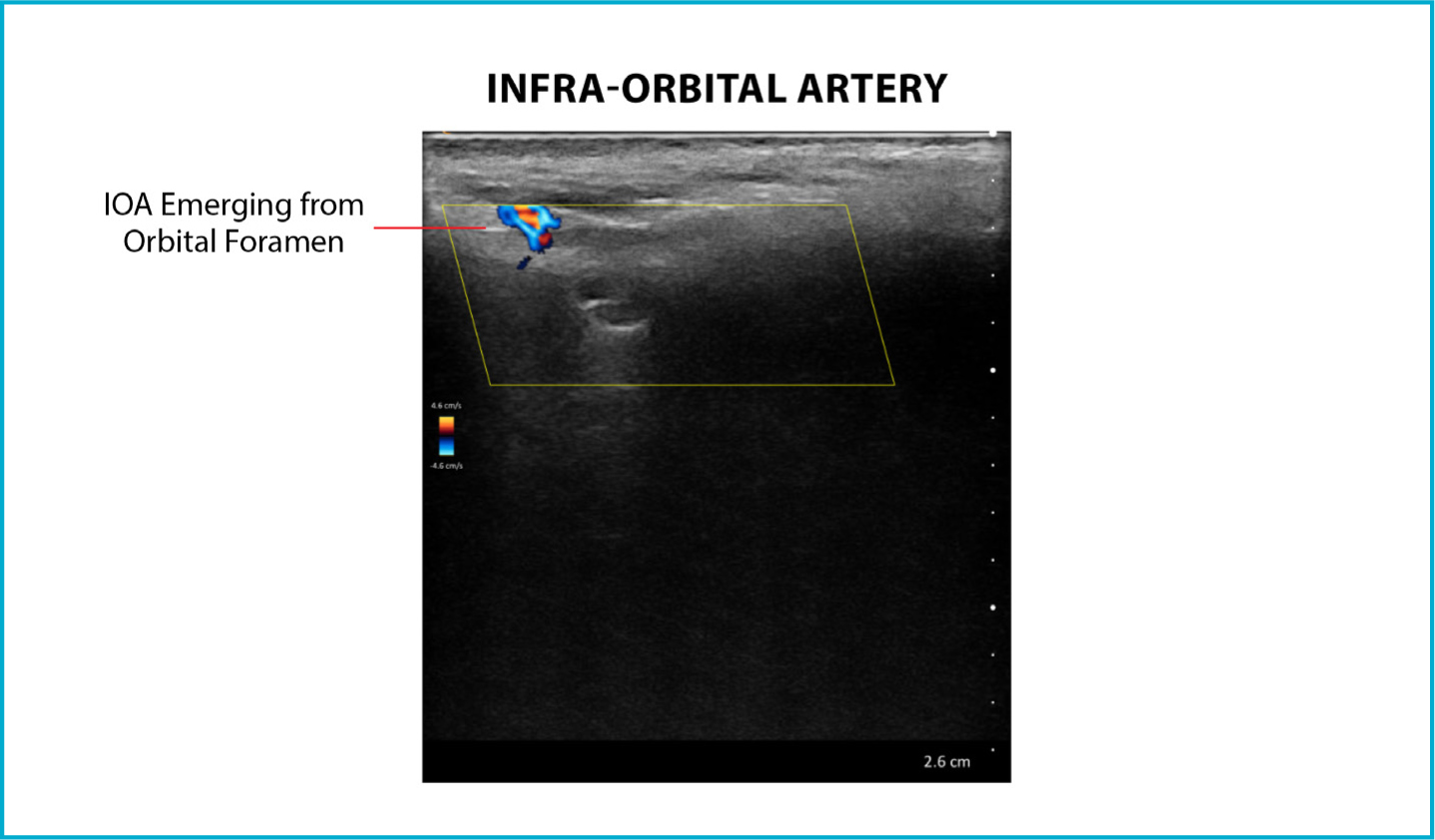
Moving to the temple, with the transducer at the hairline, the temporal bone, temporalis muscle and superficial temporal fascia can be visualized (Figure 10). As the transducer is moved medially the deep fat pad will come into view and sometimes even the temporal extension of the buccal fat pad can be glimpsed.
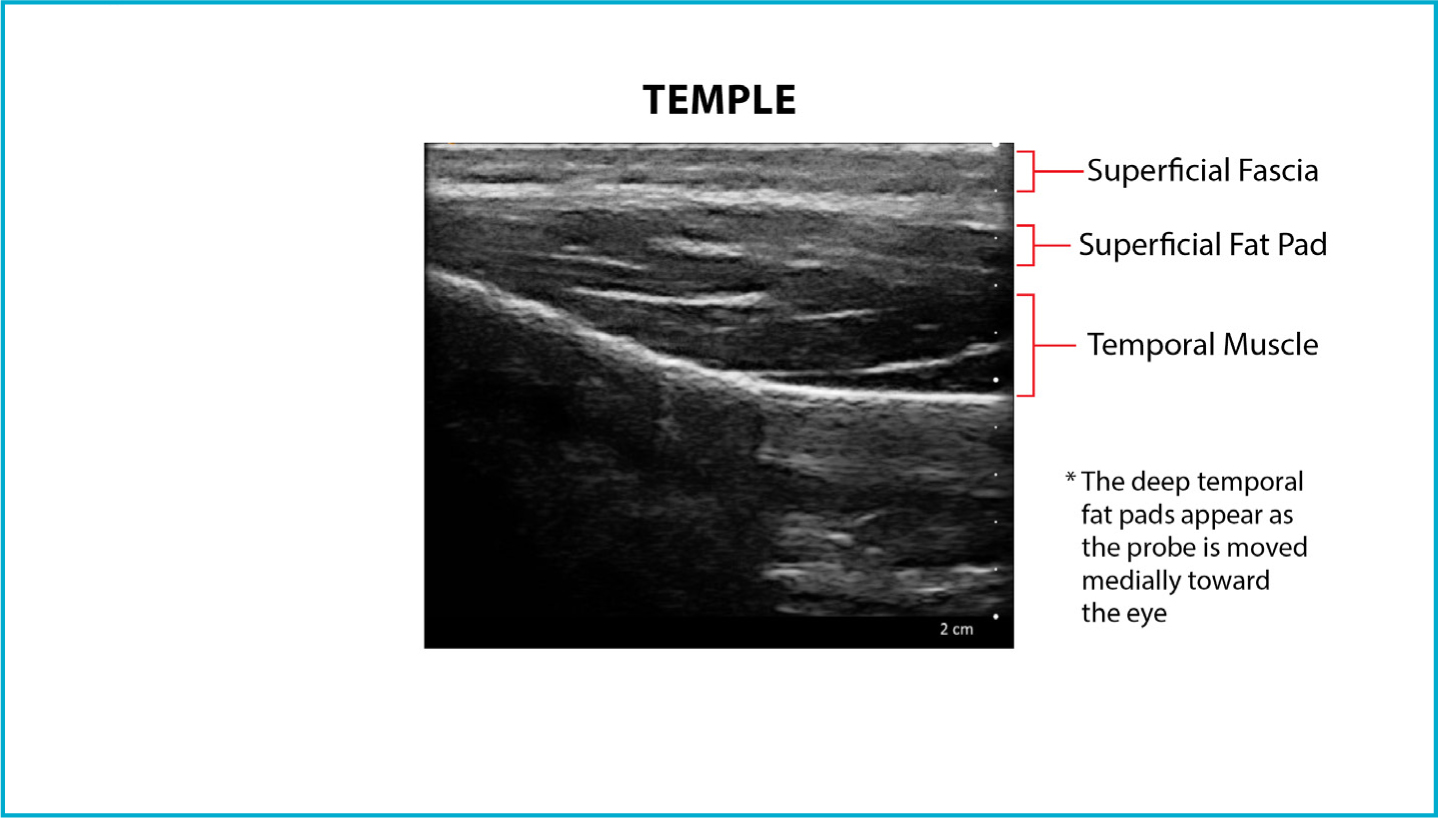
The external carotid artery travels from the neck, behind the ramus of the mandible to the anterior ear, giving off the maxillary, transverse facial and zygomatico-orbital arterial branches. It terminates at the superficial temporal artery, which divides into parietal branches to supply the scalp. The maxillary artery travels behind the condylar process of the ramus and behind the zygoma, to give rise to the deep temporal artery branches.
In contrast to the anatomic variety found in the branching of the labial arteries from the facial artery, the superficial temporal artery is more consistent in its pathway, and serious anatomical variations are relatively rare (Koziej et al, 2019). Within the temple, a superficial ‘artery-free’ zone or injection plane may be estimated by imagining a 3 cm radial line starting at the juncture of the frontal and temporal processes of the zygomatic bone (Koziej et al, 2019). Using the frontal process and zygoma as lateral boundaries, this creates an approximate quarter of a circle, ending at the zygoma and frontal process (Figure 11).
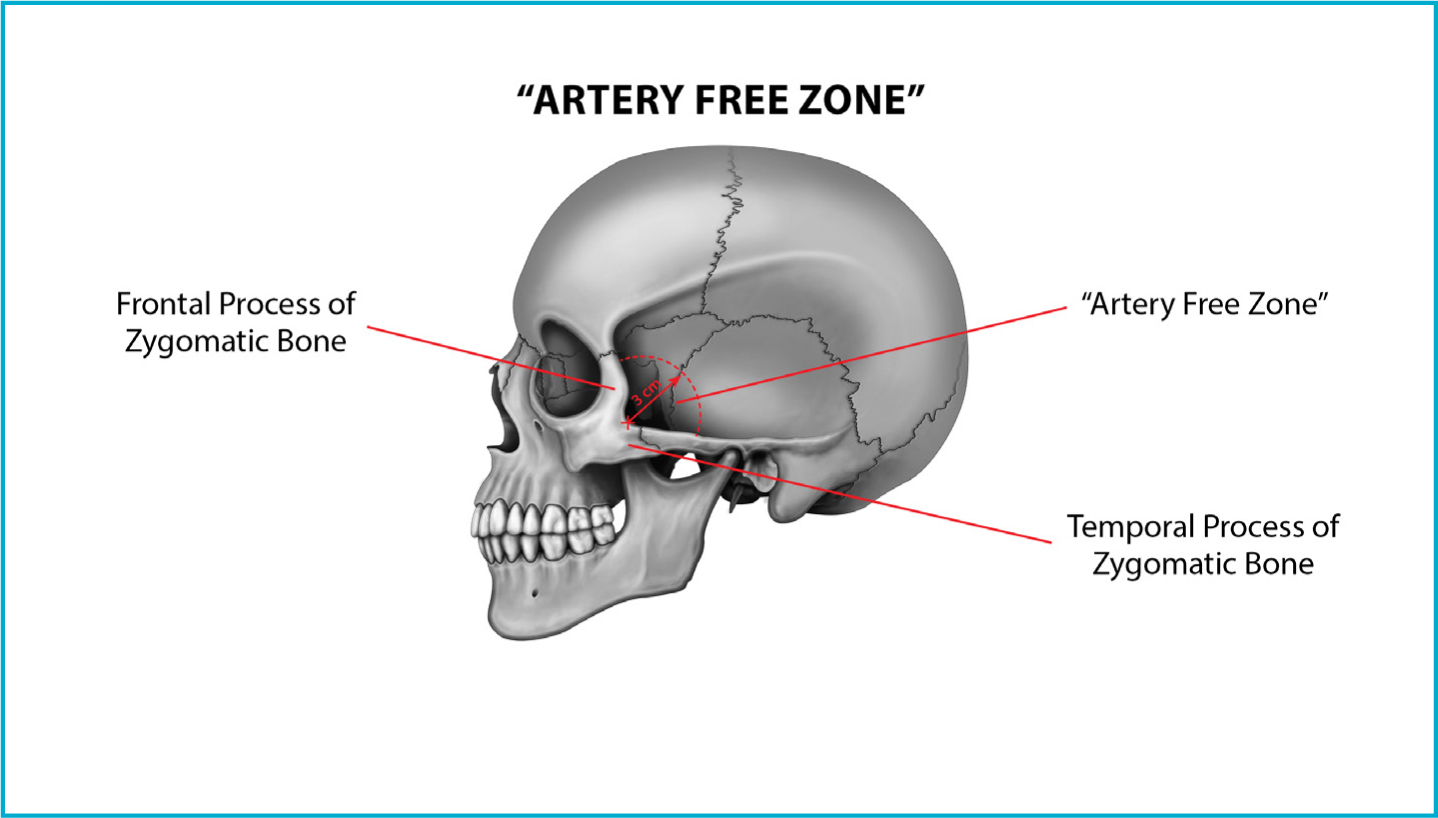
Sliding the probe from the temple region to the mid-forehead, the bright white line representing the frontalis can be observed, as well as the thin dark strip above the bone, the frontalis muscle (Figure 12). Any image below the bone is a reverberation or artefact. The highly reflective bone leads to a series of linear echoes that project below the bone itself (Velthuis and Schelke, 2022). These echoes can be ignored.
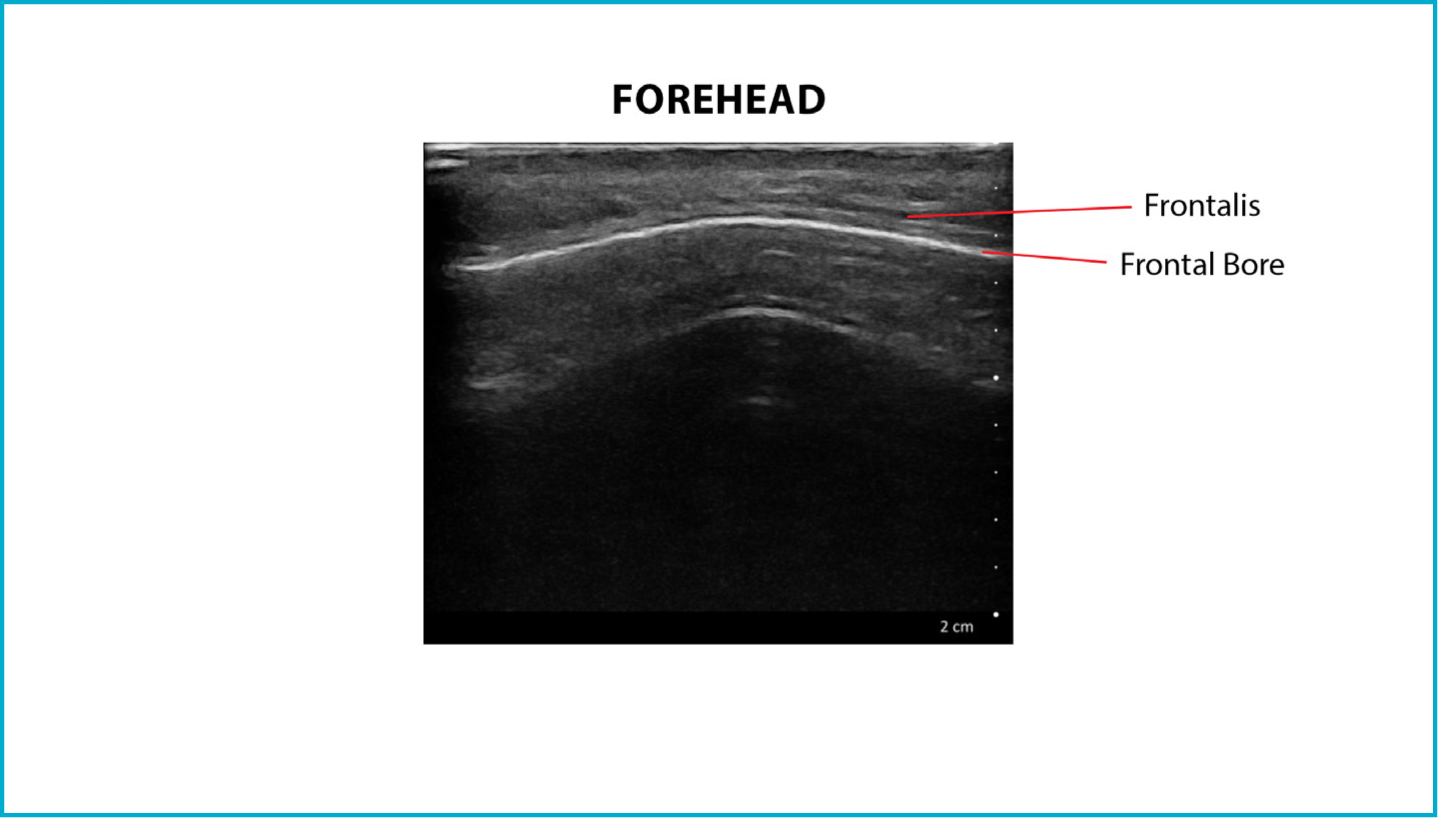
Conclusion
Learning and using facial ultrasound can be a difficult and daunting task. As with any new skill, consistency and repetition are key to becoming comfortable with this modality. Training the eye to interpret the various shades of grey and recognise vascular, fibrous and muscular structures is time-consuming, yet rewarding. The ability to use ultrasound in an emergency and prevent serious filler-induced complications is the most compelling reason, of many, to become proficient with this tool.